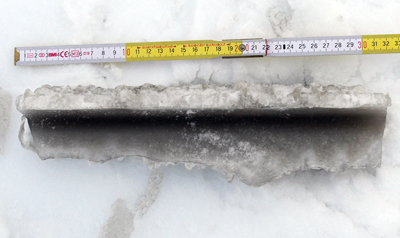
The International Energy Agency’s Wind Technology Collaboration Programme Task 19 ‘International Recommendations for Ice Fall and Ice Throw Risk Assessments’, published in October 2018, gives a comprehensive overview of the necessary parts of a risk assessment and will hopefully form the basis for a future standard. Although it was obviously created with great care, the variables involved still leave considerable leeway for the results.
By Markus Drapalik, Institute of Safety and Risk Sciences, University of Natural Resources and Life Sciences, Vienna