One problem always mentioned for offshore wind farms is the water depth and the limitation faced for turbine foundations, which leads to floating wind farms for depths over 100 metres. Although it has not yet surfaced, as wind farms get farther to sea, the distance from shore can also become a limitation for transmission.
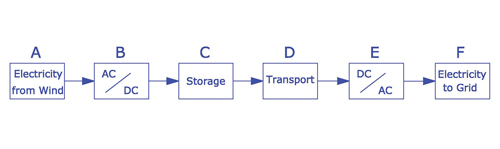
By Ahmad Hemami, McGill University, Montreal, Canada
In searching for the farthest wind farm so far, I found the following farms:
- Hornsea 1, located 120km (75 miles) in the North Sea off the coast of Grimsby, England, with a water depth of 23.3 to 31 metres, was completed in 2020 with 174 turbines and a generating capacity of 1.2 gigawatts.
- Hornsea 2, located 89km (55 miles) in the North Sea with water depths of 30 to 40 metres, started production in December 2021. When complete, it will have 165 units of 8 megawatt turbines (a total of 1.32 gigawatts of electricity).
- Dogger Bank Wind Farm, in three phases A, B, and C, each with a capacity of 1.2 GW, 130 km (81 miles) constructed in UK waters off the coast of Yorkshire. The first turbine at Dogger Bank A started turning and producing electricity in October 2023. The water depth ranges between 18 to 63 metres.
Both Hornsea’s turbines and Dogger Bank's turbines (with monopile foundations) are anchored to the ocean floor.
As can be seen, so far the distance between a farm and the shore has not exceeded 130 km, and no technical problem has been encountered. However, in the future it is quite possible that a wind farm would be developed in places that are much farther away, say 500 to 1000 km from land. On top of that, the water depth can be such that it prohibits operations on transmission cables. Although for land-based wind turbines this is not a common scenario, seeking more energy from ocean winds drags us in that direction, especially if one day wind and other types of renewable energy are substitutions for today’s gas and oil.
If transmission in the form of electric energy through cables is not possible, other options are (a) storing in mega size batteries -Battery Energy Storage System (BESS) (Figure 1), and (b) converting to other energy content media, such as hydrogen (Figure 2). In both cases, the stored energy or medium needs to be transported to shore by ship. If option (a) is employed, electricity must be converted to DC first, and then the stored energy needs to be converted back to AC at the destination. It is interesting to know that Dogger Bank wind farm uses a high-voltage direct current (HVDC) transmission line.
As can be seen, so far the distance between a farm and the shore has not exceeded 130 km, and no technical problem has been encountered. However, in the future it is quite possible that a wind farm would be developed in places that are much farther away, say 500 to 1000 km from land. On top of that, the water depth can be such that it prohibits operations on transmission cables. Although for land-based wind turbines this is not a common scenario, seeking more energy from ocean winds drags us in that direction, especially if one day wind and other types of renewable energy are substitutions for today’s gas and oil.
If transmission in the form of electric energy through cables is not possible, other options are (a) storing in mega size batteries -Battery Energy Storage System (BESS) (Figure 1), and (b) converting to other energy content media, such as hydrogen (Figure 2). In both cases, the stored energy or medium needs to be transported to shore by ship. If option (a) is employed, electricity must be converted to DC first, and then the stored energy needs to be converted back to AC at the destination. It is interesting to know that Dogger Bank wind farm uses a high-voltage direct current (HVDC) transmission line.
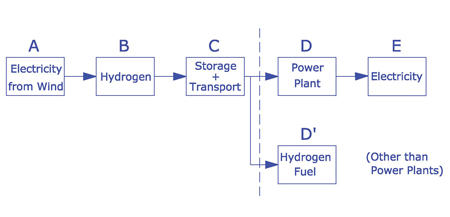
For option (b), to convert the energy back to electrical form either hydrogen can be used as a fuel in conventional power plants or it can be used in mega size fuel cells. In all cases, the efficiency of the process comes into effect and the end result is an additional cost that increases the price of the generated electricity. It is obvious that if fewer processes are involved, the more efficient is the overall operation and the lower is the additional cost. Specifically, it is realised that option (b) is better to be selected only for other applications of fuel rather than using hydrogen to generate electricity, because this implies electricity at a much higher price. For example, using hydrogen as a fuel for transportation (cars and trucks) or for heating in industrial or domestic applications is much more efficient than using it in a gas turbine or steam turbine to make electricity.
To put a price on the electricity produced using each option is not an easy task. For option (a) the facilities to convert AC to DC and those to receive electricity from special BESS vessels and convert to AC at grid voltage add their cost, to which the transportation must be added. Likewise, for hydrogen transport special vessels devoted to this purpose (high pressure or liquid hydrogen) are required.
Based on available estimations [1] to date, the cost of green hydrogen (using renewable energy) from electrolysis is between US$2 and US$6, depending on the region and the scale of operation. Then storage and transportation costs are on top of that. Finding even a very rough estimation of transportation costs also proves to be not an easy task. I thought of using the ratio between the volume of fuel usage to the volume of fuel that the oil tankers carry as an index. However, these vessels consume marine fuel that is normally heavy fuel oil (HFO), and their consumption depends on their speed. Thus, their fuel cost (per hour, for instance) cannot be expressed as a percentage of their cargo value. This implies that, again, each case must be treated based on its particular conditions at the time to come.
Further Reading
- DOE Hydrogen and Fuel Cells Program Record, cost of electrolytic hydrogen production: https://www.hydrogen.energy.gov/docs/hydrogenprogramlibraries/pdfs/20004-cost-electrolytic-hydrogen-production.pdf?Status=Master